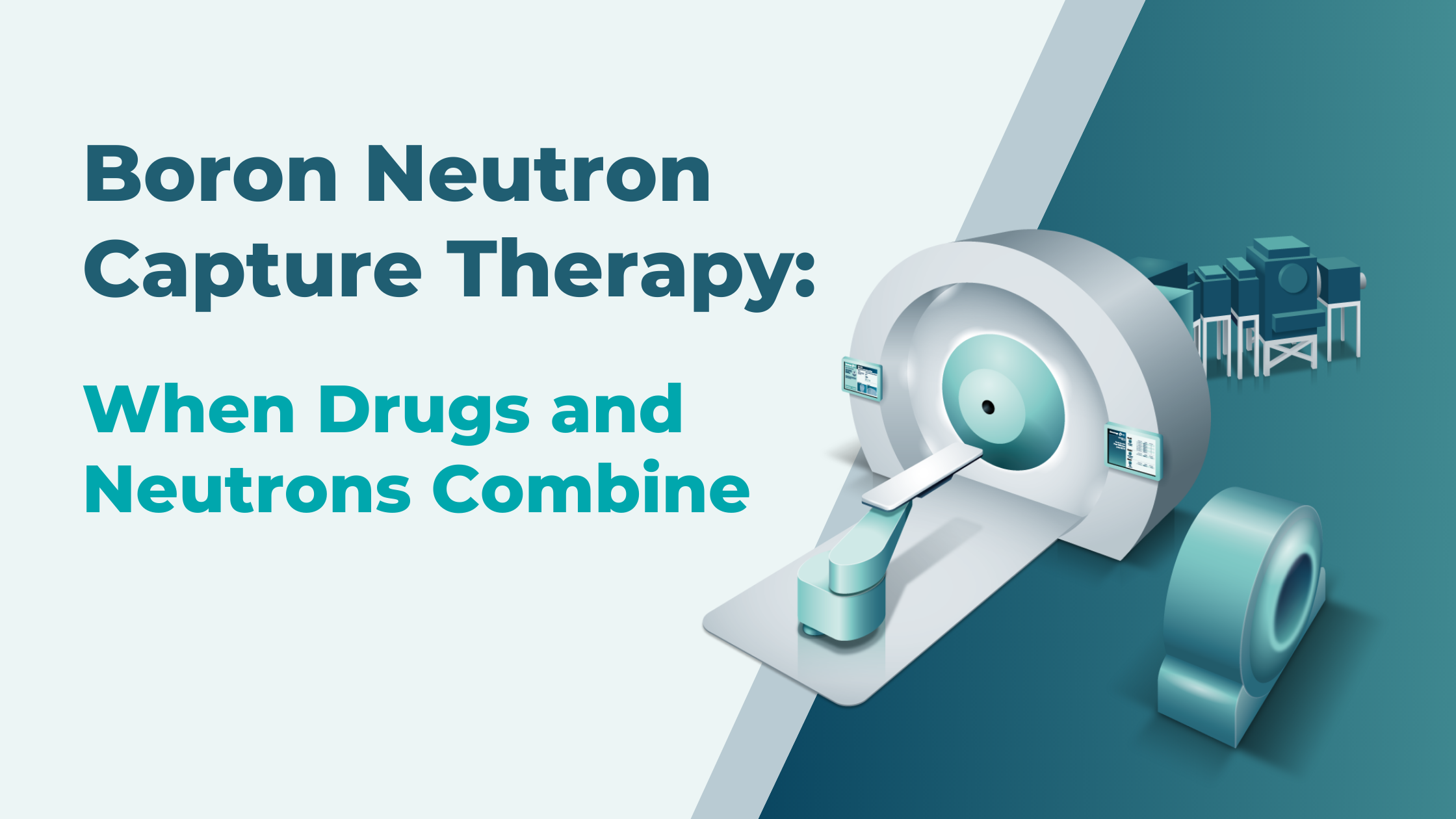
Boron Neutron Capture Therapy: When Drugs and Neutrons Combine
Oncologists must deliver conventional radiation therapy in as many as thirty to forty treatment appointments to prevent significant side effects to the patient. By contrast, BNCT requires only one or perhaps two 20-minute to 30-minute hospital patient treatments. The latter could be ground-breaking in the active lives of many cancer patients.
A single BNCT installation has the potential to treat as many patients for cancer as up to eight current linear accelerators. This would suggest significant savings on shielded room utilisation, number of staff, and device maintenance for Radiation Oncology departments.
In the next two decades, the global opportunities are enormous for hospitals and manufacturers of compact BNCT devices, software, and delivery drugs.
Estimates show that at least 1,800 BNCT devices could be operating in oncology departments worldwide in twenty years to meet global demand. Financing these compact accelerator-based devices and boron-based drugs will be one of the most compelling investment opportunities in the radiation therapy market.
Boron Neutron Capture Therapy — an old treatment friend, with new technology
The European Commission projects that by the year 2040, cancer cases will increase by forty per cent. Studies predict similar surges in cancer for the US and Asia. However, cancer mortality and morbidity levels are projected to remain almost unchanged.
What could be the Holy Grail for the radiation oncologist in light of this?
- Will it be a treatment modality with which the oncologist has to treat the patient only once or twice instead of in dozens of fractions?
- Will it be a radiation therapy that can use high radiation doses that kill just the tumour cells?
- Will it be a therapy that won’t make the patient feel progressively sicker with treatment side effects?
One such solution is boron neutron capture therapy (BNCT), the hybrid modality that combines neutron radiation sources and boron delivery drugs. BNCT has been known to researchers since 1951, but studies and clinical development were not plentiful in past years as it demands thermal neutrons. For decades, the only source for these relatively slow neutrons were nuclear fission reactors that are typically located far from hospitals.
Sending scores of patients to a nuclear power plant each day was obviously neither a practical nor an appealing clinical practice. But recent advances in linear accelerator development are fueling the revival of boron neutron capture therapy.
In Japan, for example, treatment in fully-functioning BNCT clinics is already approved for reimbursement by Japan’s national health insurance system. In Europe, the BNCT centre at the Helsinki University Hospital with a room-sized proton accelerator is slated for clinical trials in 2022.
The physics of boron neutron capture therapy
BNCT combines two components and does so in two steps: infusion with boronated agent and irradiation with epithermal neutrons. It joins the biological selectivity of cells with radiation power, targeting tumour cells without causing radiation damage to the adjacent healthy tissue.
Boron neutron capture therapy is a radio-therapeutic modality based on the nuclear reaction .
Before the treatment fraction, a nurse administers an intravenous infusion of a delivery drug containing the non-radioactive 10B isotope to a patient.
The 10B atoms collect in the patient’s cancer cells to capture the low-energy neutrons coming from the BNCT device in a treatment room. The 10B (boron-10) atoms instantly decay into an alpha particle (4He), a recoiled lithium nucleus (7Li), and a γ particle.
In the immediate proximity of the reaction, both the lithium nuclei and alpha particles deposit their energy over distances less than 10 μm, which is within the diameter of one cell — the cancerous one.
Delivering Boron-10 to the diseased cell
Now that we have accelerator-based sources of the correct epithermal neutrons, research is intensifying in the second modality of BNCT — the delivery and selective absorption of the boronated agents in human tissue.
The requirements are high for the boron-10 carrier compounds. They have to provide:
- low toxicity;
- high tumour uptake;
- low accumulation in healthy tissue;
- quick clearance from the patient’s tissue and blood after treatment;
- at least three times higher 10-boron concentration in tumours than in normal tissue, and
- constant and equally spread in-tumour concentration during BNCT irradiation.
Historically in BNCT, boron-10 has been delivered typically with two agents, sodium borocaptate (BSH) and boronophenylalanine (BPA). The latter is sometimes complexed with fructose to be more soluble (BPA-F).
Today’s most common therapeutic drug used in BNCT is BPA.
But these boron-10 compounds have a hard time fulfilling all the mentioned requirements for delivery and functionality. The good news is that there are several new boron-10 delivery substances on the research horizon!
Biological Boron-10 compounds: the final frontier
Biochemists and biologists are racing to develop third-generation boron-10 delivery agents that will take full advantage of the optimised neutron beams produced by compact accelerator-based BNCT devices.
Researchers are taking pages out of the “handbook” for developing and evaluating modern substances for targeted biological therapy, for example, utilising low and high molecular-weight compounds as boron-10 carriers. As these compounds show greater specificity for cancerous cells, clinicians will be able to lower boron-10 treatment concentrations.
In parallel, work is continuing in improving the dosing and delivery of the well-established BPA and BSH agents.
Three hours in the life of a BNCT patient
So, how does a typical fraction with boron neutron capture therapy look to a patient?
In the preparation room of the outpatient BNCT clinic at a hospital, a nurse administers the boron-10 isotope delivery compound to the patient by intravenous infusion. The patient rests while the delivery agent accumulates in their cancerous cells.
At intervals, the nurse measures the patient’s blood-boron concentration, usually with a plasma optical-emission spectrometry device.
When the absorption values are sufficiently high, generally after a couple of hours, an oncology technician takes the patient to the treatment room and places them lying down on the robotic positioning couch in front of the BNCT’s beam shaping assembly.
To position the patient accurately before treatment delivery, the technician performs imaging with the in-room CT scanner for correlation with the planning images,
which increases treatment accuracy.
Irradiation with neutron beams follows. The epithermal neutrons react with the boron-10 substance accumulated in the patient’s cancer cells and selectively destroy them while leaving the healthy surrounding tissue intact.
Modern BNCT has a very slight impact on the patient’s quality of life – the smallest of all other radiation, chemotherapy or biological therapy modalities. And the fact that at most two fractions are enough means the patient does not have to be returning to the clinic for weeks and months. BNCT is therefore very suitable for recurring cancers where all other treatments are not permissible.
Boron neutron capture therapy will be in almost every hospital
Therapy directly in the oncology ward is possible because today’s BNCT devices can be very compact. For some models, the equipment of the specialised neutron source fits into a small shielded hospital room.
The BNCT device consists of a proton accelerator, a beam transport system, a solid lithium target for neutron generation and a neutron circular-beam shaping assembly. The device also has online monitoring systems for the proton and the neutron beam.
Usually, radiation oncologists perform simulations based on CT, PET and MRI images
to perform treatment planning for BNCT. The critical advantage of BNCT is that tumour marking is achieved biologically and not geometrically with a 3D model of the scanned image as in conventional radiation therapy.
Plan shortness is crucial from both the financial perspective (higher throughput of patients) and the comfort perspective of the patient being treated. There is a vast difference between lying still on a treatment couch for 20 minutes versus an hour.
On the other hand, the neutron beam in BNCT is stationary, so patient positioning may need to be more elaborate than in conventional radiation therapy. Sometimes positioning demands several couch settings to cover all treatment areas, but this is not inconvenient for the patient.
The broader potential of BNCT treatment
Based on several decades of studies, boron neutron capture therapy is capable of treating almost twenty per cent of all new cancer cases. That is without considering all the unique biological delivery agents for boron-10 that are entering clinical testing as we speak. These will further decrease potential toxicity and improve the efficacy of BNCT.
Experts estimate that by 2040, at least 5.4 million patients worldwide will be suited for BNCT. The global opportunities are enormous, both for manufacturers of BNCT devices, software and delivery compounds — and for oncology departments of hospitals everywhere.
As of now, BNCT has been clinically studied mainly on non-curable recurrent cancers, with a high mortality rate and limited treatment options.
As modern clinical oncology is continuously looking to increase the therapeutic ratio,
proponents of BNCT are already exploring the possibility of other curative treatment regimes. These could cover a much more comprehensive range of disease sites and tumour types.
Typically, radiation therapy has been used to treat localised tumours, while chemotherapy was applied body-wide. But BNCT targets cancer on the biological level and opens possibilities for irradiating large volumes of the body to deal with metastatic cancers.
More clinical studies have to be performed to evaluate metastasis treatment, but on theoretical grounds, it is promising. Indeed, early-adopter clinical sites might soon be combining BNCT with existing oncological methods and therapy techniques, such as chemotherapy, surgery, and conventional or proton radiation therapy.
Market potential of boron neutron capture therapy
There is a substantial global market opportunity for new cancer treatment modalities. The world’s population is ageing, especially in developed countries.
The data on BNCT’s resurgence as an accelerator-based solution is impressive:
- six industrial BNCT manufacturers;
- six scientific-research BNCT initiatives;
- 16 BNCT centres operating at different development phases;
- 20+ BNCT centres planned globally;
- 10 active BNCT delivery compound developers.
At least 1,800 BNCT devices could be operating in clinical wards worldwide in twenty years. Investors into manufacturers of BNCT devices, which typically sell for about $25 million, are excited.
Several BNCT device types are currently nearing their regulatory approval. Once they acquire it, many analysts believe the BNCT market will really take off.
But a BNCT device costs perhaps eight times more than an X-ray LINAC for photon therapy, so where is its economic advantage?
Firstly, oncology wards can potentially treat eight times more patients with a single BNCT installation than with current X-ray devices, as boron neutron capture therapy needs on average only 1.5 daily fractions per patient.
The time needed to complete a typical BNCT fraction is 30 minutes, including patient positioning, implying that one device could treat up to 16 patients in one shift. Accordingly, hospitals need fewer shielded rooms, reduced staff and less costly device operational expenses.
Considering one BNCT device can conceivably perform the work of roughly eight X-ray (RT) therapy devices, the cost of BNCT per treatment could be pretty close to RT when used at total capacity.
What lies ahead for BNCT?
The future looks pretty bright for boron neutron capture therapy.
BNCT can deliver high treatment efficacy to oncology clinicians while ensuring short treatment time resulting in greater patient comfort during treatments, fewer side effects and faster recovery.
BNCT has been clinically evaluated on treatment-resistant tumours in the most inaccessible anatomical locations. There are, of course, still many advances to be achieved to enhance the modality’s therapeutical value further and optimise the technology for higher-volume device manufacturing.
Besides the new compact accelerator designs and the fruits of accelerated research in biological delivery boron-10 compounds, it will be innovations in software that will bring BNCT to all large hospitals and oncology wards as an efficient and economical new therapeutic cancer modality.
And perhaps, boron neutron capture therapy will, in combination with other treatment modalities, finally start winning the battle against cancer.
To learn more about boron neutron capture therapy, join our upcoming educational webinar on March 2nd 2022: Boron Neutron Capture Therapy – Today’s Use and Future Aspects.
More on BNCT
If you want to learn more about Boron Neutron Capture Therapy, read our other blogs on the topic, or watch our webinars.
Ian Norton has worked in Medical Physics and Radiation Oncology for over 20 years, previously with the University Hospital of Zurich and until recently with Philips Healthcare. He is now the Strategic Sales Manager for Healthcare at Cosylab. He has expanded the use of innovative new approaches to Radiation Oncology in many hospitals and clinics worldwide. In his free time, he enjoys hiking and skiing in the mountains.