The Future of Radiation Therapy with Online Adaptive
In October 2021, we participated in a symposium that took a measured look at current takeaways and future possibilities of online adaptive radiation therapy. Stephen Towe, CEO of Leo Cancer Care, moderated the online event. The panel included Niek Schreuder and Thomas ‘Rock’ Mackie from Leo Cancer Care, Nikos Paragios from TheraPanacea, Fritz DeJongh from ProtonVDA and Uros Mitrovic from Cosylab.
It is already clear that radiation therapy will be enhanced by faster computation, better and streamlined workflows, and improved imaging to handle higher patient throughput, various modes and specialised hardware. The latter will also enable online adaptive technology to become economical.
Using proton-beam imaging, we will achieve more efficient and safer online alignment and verification of a patient in the treatment position. The overall cost of therapy planning will be lower with proton imaging, allowing for more precise treatment. And artificial intelligence (AI) will streamline the whole treatment workflow, enabling clinical teams to be more effective and less overwhelmed.
Improved computation, workflows and imaging
According to Rock Mackie, all the technology drivers are now coming together. We are beginning to have enough computing power to do all the calculations needed. We are also proceeding with the correct algorithms and have developed the hardware to perform the required imaging in real-time and specialised modes.
A few years ago, offline adaptive radiation therapy consisted of treatment planning and quality assurance. If you found your patient was deviating after you started a course of treatment, you had to perform replanning, which is a serious and time-consuming task. In Rock’s opinion, online adaptive is all of the above, but on steroids. You have to integrate during the current treatment, quickly and not in a couple of days; you have to do this in real, or nearly real, time.
Rock believes now is the time to rethink setting up the patient for treatment. Are they resting and prone, or do we put them in a seated, semi-standing or perched position, which can in some instances be optimal? The upright positioning has several advantages, such as facilitating quick imaging and patient setup. Artificial intelligence will undoubtedly drive most of these procedures, also in the pre-therapy phase.
“The challenges are algorithm development, high-speed computing, and the hardware to fully optimise how the patient should be treated and imaged at the time of treatment.”
Why use an upright patient position during therapy?
Niek Schroeder underlines the radiation therapy benefits of combining the treatment and imaging of patients in an upright position. A CT (computed tomography) imaging device, mounted on a ring, scans the patient quite near the isocentre position. Of course, if the tumour is off-centre, the patient has to be shifted slightly after the imaging.
By adding a CT ring around the isocentric position of the device, we acquire direct measurement of the patient’s anatomy, with all the parameters, to which we can apply the AI methods to verify the tumour and organ positions and check whether everything is correct.
Niek sees three additional advantages of an upright patient being rotated. One is that more precise and reproducible imaging is possible if both the beam and the imaging device are stationary. The second usefulness is that turning the patient on a rotating platform borne by a massive bearing gives us extreme isocentricity, much higher than in systems that rotate a beam around the patient. And the third benefit of rotating an upright patient is much more space around the patient being available for positioning a device for image guidance, which means we can use CT and pioneering technologies such as proton radiography.
“With online adaptation, we can detect anatomical changes and adapt to the daily situation. Clinicians can use therapy plans that wouldn’t be robust under today’s uncertainties with standard, offline adaptative therapy.”
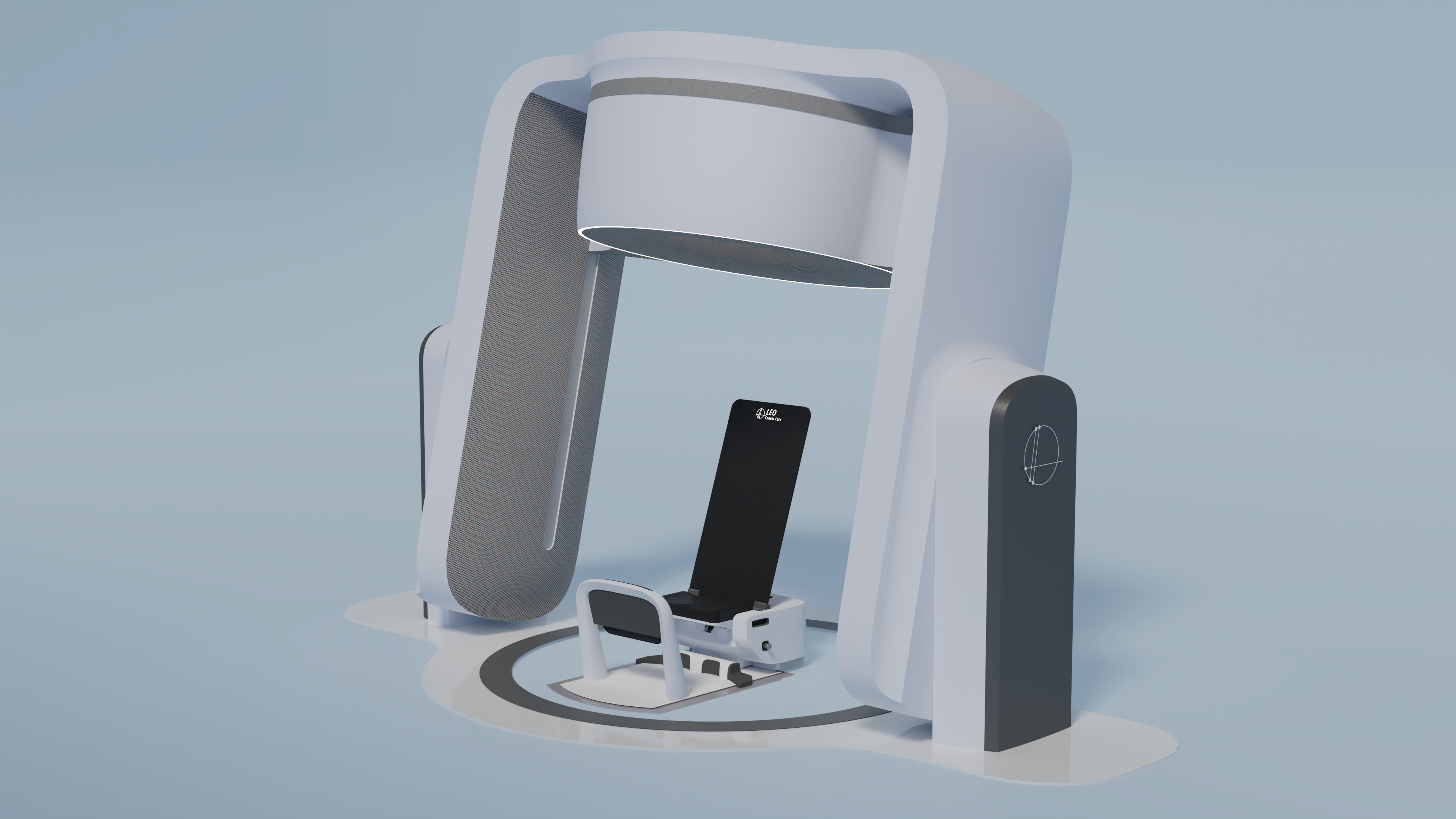
How does proton imaging increase the effectiveness of proton therapy?
In proton therapy, oncologists currently use the data of pre-therapy CT images to calculate the proton relative stopping power (RSP) and deduce the proton range in the patient. Using this range, they create the treatment plan. The latter, however, has additional range uncertainties and larger target margins due to data conversion.
Fritz DeJongh describes in detail his team’s clinical experience of using proton imaging to quantify RSP directly. A group of researchers working with the Northwestern Medicine Chicago Proton Center compared the directly-determined proton RSP values with the ones recalculated from CT images, using both cylindrical phantoms with tissue-equivalent inserts and cadaver phantoms.
The results showed that the most significant accuracy increase of RSP, based on proton imaging, was achieved for cavitation areas and dense bone. Furthermore, the measured imagining doses were 10 to 100 times lower for proton imaging than for CT imaging. The latter could enable frequent proton imaging before each treatment fraction, with negligible adverse effects on the patient.
Fritz believes in the benefits of online adaptive radiation therapy and is enthusiastic about using proton imaging for planning proton therapy for upright-positioned patients. With proton imaging, online adaptive can perform more exact proton relative stopping power calculations, more accurate proton treatment plans and much narrower beam-fields while providing more conformable treatment.
“What is now lacking in radiation therapy is software which can exploit the latest hardware advances. Areas such as conducting workflows for various radiation therapy trials are still heavily reliant on human expertise.”
Can deep learning speed up the contouring of organs?
Nikos Paragios observes that radiation therapy progressed hugely on the hardware side during the last few decades, especially devices for initial planning. But what is now lacking is software that can exploit the latest hardware advances.
Areas like conducting workflows for various radiation therapy trials are still heavily reliant on human expertise. Therefore they cannot be used for online adaptive, simply because we don’t have sufficient time to complete the job. Furthermore, even most of the West’s “standard” radiation-oncology solutions that demand significant human expertise cannot be used in developing countries where the expertise is lacking.
And because we do not do perform online adaptive radiation therapy today, most treatments are associated with high-toxicity risks. To address these issues, we have to develop software solutions for treatment planning, such as automatic delineation, that are based on artificial intelligence.
Deep learning solutions can already speed up and standardise the contouring of organs, based on CT or MRI, to automatically annotate, for example, in a few dozens of seconds more than 150 structures in the brain.
Advanced software can generate a fully planning-compatible synthetic CT within seconds from MRI images. Soon, AI-based treatment planning solutions will automatically determine the specific constraints that can be used for a patient given the tumour’s location and the physician’s prescription.
“The role of artificial intelligence is to streamline the workflow and minimise user interaction. By having AI tools execute the adaptive process, we will also need AI methods to automate the quality assurance of the process.”
Artificial intelligence should streamline the whole treatment workflow
Uros Mitrovic illuminates the importance of the treatment control system being flexible. As we know, the online adaptive subsystem carries out the processing, assessment of the anatomy change, and replanning. Once this exercise is completed, the adaptive plan is sent back to the treatment control system, and the workflow continues in a regular manner.
Uros sees the advantage of the treatment control system being designed in such a fashion as to support working with the original and the adaptive treatment plan interchangeably. It is a no brainer that the treatment control system will, eventually, perform the dose-driven image guidance in the treatment room and automatically calculate the treatment plan, on the fly.
However, we should not forget the importance of plan approval, as we usually have a very rigid set of boundary conditions. Before we treat a certain fraction, we must ensure all the parameters are within acceptable limits. It is good to have a lot of information, but we have to remember that we have a solid benchmark — the existing plan. We can set boundaries such as being allowed to go up five per cent in monitoring areas, and, for example, have three per cent less energy, and so forth.
As the radiation therapy industry, we have been through this before, when we started imaging patients before treatment. For instance, we brought in the ability to shift the table, and we defined boundaries regarding how much table shift is acceptable for effective work.
The role of artificial intelligence in treatment planning is to streamline the workflow and minimise user interaction. We observe on the clinical side that, naturally, teams do not welcome having to perform more work. Therefore, by having artificial intelligence tools execute the adaptive process, we will also need AI methods to automate the quality assurance of the process. Only with these two steps working together in an automated fashion can we prevent additional work-time intruding into the patient workflow for the clinical staff.
What lies ahead for online adaptive radiation therapy
The future of radiation therapy is certainly adaptive. There is probably not just one solution regarding the type of imaging used to support online, or even real-time, adaptive. With proton radiography, you directly measure the proton’s stopping power and compare it with what you’ve assumed for the proton-therapy treatment planning, thus achieving optimal treatment.
One of the main takeaways from testing online adaptive radiation therapy is that a lot revolves around data collection. The more information we can get directly using the proton beam, for example, the better our modelling will be and the superior the adaptation results.
Artificial intelligence will rationalise all treatment workflow and give doctors and clinical staff the tools to increase effectiveness and spend less time on repetitive procedures, increasing the focus on individual patients.
If you’d rather listen to the above, watch the video clip from the symposium:
ABOUT THE PANELISTS
Thomas Rockwell (“Rock”) Mackie, PhD is Emeritus Prof. in Medical Physics, Human Oncology, and Engineering Physics at the University of Wisconsin-Madison and the Director of Medical Devices Focus Area at the Morgridge Institute for Research. He is a medical physicist that also developed a safer type of radiation therapy called tomotherapy, which produces less radiation without lowered effectiveness and is the marriage of a linac and a CT scanner. Dr Mackie is also focused on the development of a compact proton therapy device.
Nikos Paragios, PhD, is the principal founder of TheraPanacea and an artificial intelligence researcher who has worked on many research subjects that straddled both AI and oncology. He noticed an immense gap between the technologies used in hospitals and the technological and scientific advances achieved in research. He founded a startup to bring the most recent discoveries in AI faster into the clinical arena.
Fritz DeJongh, PhD, is a co-founder of ProtonVDA. With a PhD in Physics from Caltech and 22 years of experience as a physicist at Fermilab, Fritz DeJongh studied the clinical needs and constraints that were not being addressed by long-term academic programs and developed several concepts aimed at addressing these needs in a fast and cost-effective way, which serve as the basis for ProtonVDA.
Niek Schreuder, PhD, is a board-certified medical physicist with significant experience in all aspects of radiation therapy (RT) and in managing medical-physics teams and training medical professionals in RT. He is currently President of Proton Therapy at Leo Cancer Care. Niek is respected in the PT community as an engineer of innovative solutions for standardising and simplifying PT facilities to reduce the costs and time-to-market.
Uros Mitrovic, PhD, is an industry expert with over ten years of experience in several medical device fields, including radiation therapy and medical imaging. He holds a PhD in medical image processing and is the author or co-author of several papers in prestigious journals, conference proceedings and US patents. At Cosylab, Uros is the Marketing Director and the Portfolio Manager of the OncologyOne product suite.