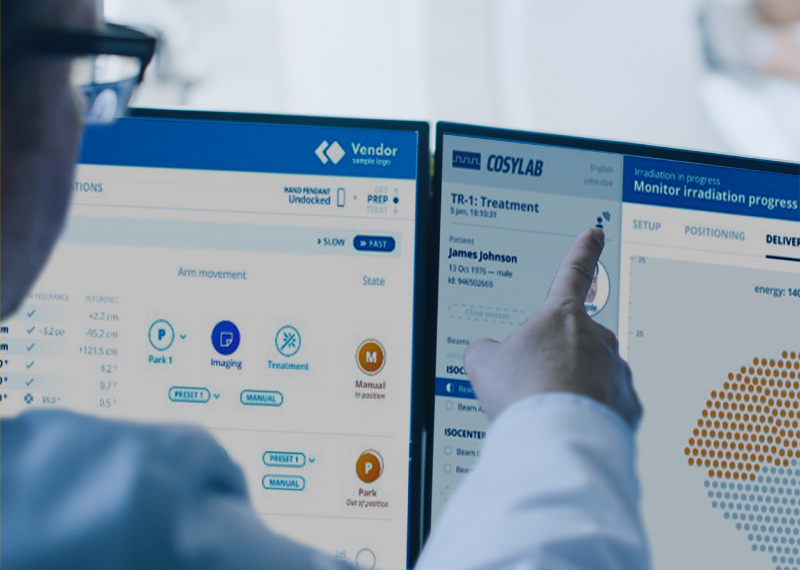
The RAPTOR Project: How to Create a Real-time Tumour-eating PT Beast
The advantages of particle beams over X-rays in oncological therapy are well known, and I believe most of us know about them. The problem is that in most clinical practices today, the inherent accuracy of proton therapy is not leveraged to its fullest, opening the door to speculations regarding its cost sustainability.
On the 1st of March 2021, the Horizon 2020 Programme »RAPTOR« will officially commence and start collecting applications from PhD candidates who would like to participate in one of the most advanced radiotherapy fields. RAPTOR aims to bring adaptive particle therapy to the clinic, with real-time treatment planning, and prove that it is value-based and economical.
So, what is hiding behind the RAPTOR acronym?
FIRST, THE RAPTOR THING
RAPTOR stands for Real-time Adaptive Particle Therapy Of Cancer and is a groundbreaking and multidisciplinary project funded by the European Union.
Besides Cosylab, fourteen other organisations cooperate in the European Horizon 2020 project consortium whose purpose is to perform research both at academic and non-academic health care facilities. RAPTOR will also recruit fifteen young researchers through the Innovative Training Network (ITN) of the Marie Skłodowska-Curie scheme to work at the consortium partners. The latter include universities, research centres and companies.
SECOND, PT AND RT
Particle therapy (PT) is external beam radiotherapy that does not use X-rays as “classical” radiotherapy does, but beams of energetic protons, or other heavier positive ions. The most prevalent PT for cancer treatment is proton therapy. Oncologists also employ treatment with Carbon-ions (C-12); however, the medical machines that utilise the latter are much more complicated than proton-based systems and thus are less available. PT machines speed up ions in specially designed accelerators. Because of the different nature of charged particles to photons, PT machines are larger and more complex than LINAC (linear accelerator) devices for X-ray therapy. PT machines are therefore more expensive to construct, have higher running costs and demand more operational effort.
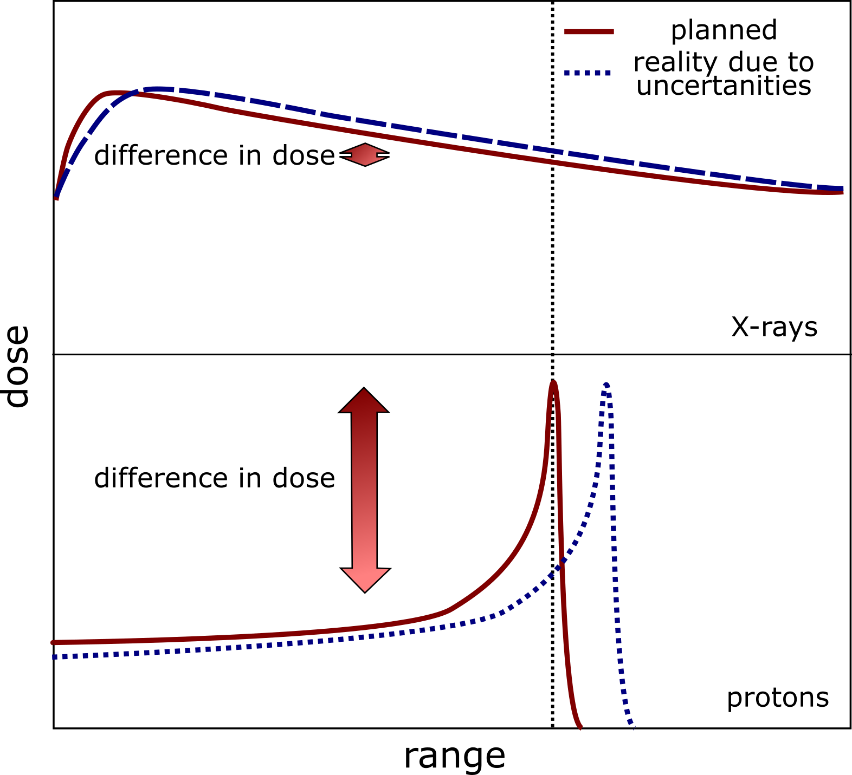
Figure 1 – Schematic representation of the effect of position uncertainties on dose deposition. A small shift in the Bragg peak can result in a significant dose difference to both healthy and malignant tissue.
On the other hand, PT has advantages when compared to traditional X-ray therapy. The latter deposits the radiation dose in tissue with approximately exponential decay, while PT deposits most of its dose in the region specified by the Bragg Peak. The more we can determine the precise three-dimensional position of the tumour over time, the better the particle beam can be energised and shaped to irradiate the whole tumour. Hence, the more exact the beam-shaping is in PT, the less it damages healthy tissue surrounding the cancerous growth.
LOOKING AT COSTS
So, why do radiation oncologists treat such a low percentage of cancer patients with PT – only about five per cent globally? One reason is the current cost and availability of medical-accelerator facilities needed for PT. The other reason is that the high precision of proton therapy appears as a double-edged sword. On the one hand, PT is extremely precise, but we have to know precisely the shape and location of the targeted tumour.
Regarding the cost, we are still caught in the classical chicken-or-egg causality dilemma. PT has not yet reached the economy of scale where the price per therapy would start dropping significantly. On the other hand, for an increase in the annual number of built PT centres, the rationale of lower costs has to be validated. The price of one therapy session of PT will always be higher than that of a photon therapy session. But we have already mentioned the clear physical advantages of PT over therapy with X-rays. Can we optimise these benefits so that the higher price of PT sessions would be mitigated by the lower total healthcare costs of curing a patient in the long run?
In fact, we can – by using real-time adaptive technology to utilise the high precision of PT to the fullest!
JUST-IN-TIME TREATMENT PLANS
Today, at each PT session, the oncologists irradiate the patient a couple of days or even a whole week after they had imaged the tumour and surrounding tissue with a CT scan. The doctors base their treatment plan on the initial scan – using a past image to perform a treatment in the present.
Patient anatomy changes daily, even hourly, due to breathing patterns, bowel movements, filling the bladder, and nasal cavities. These uncertainties, combined with several others, can significantly displace the location of the pre-calculated Bragg Peak of the beam away from the exact and current anatomical location of cancerous growth. The latter severely reduces the PT treatment session’s success, as the Bragg Peak of the particle beam partially “misses” the tumour.
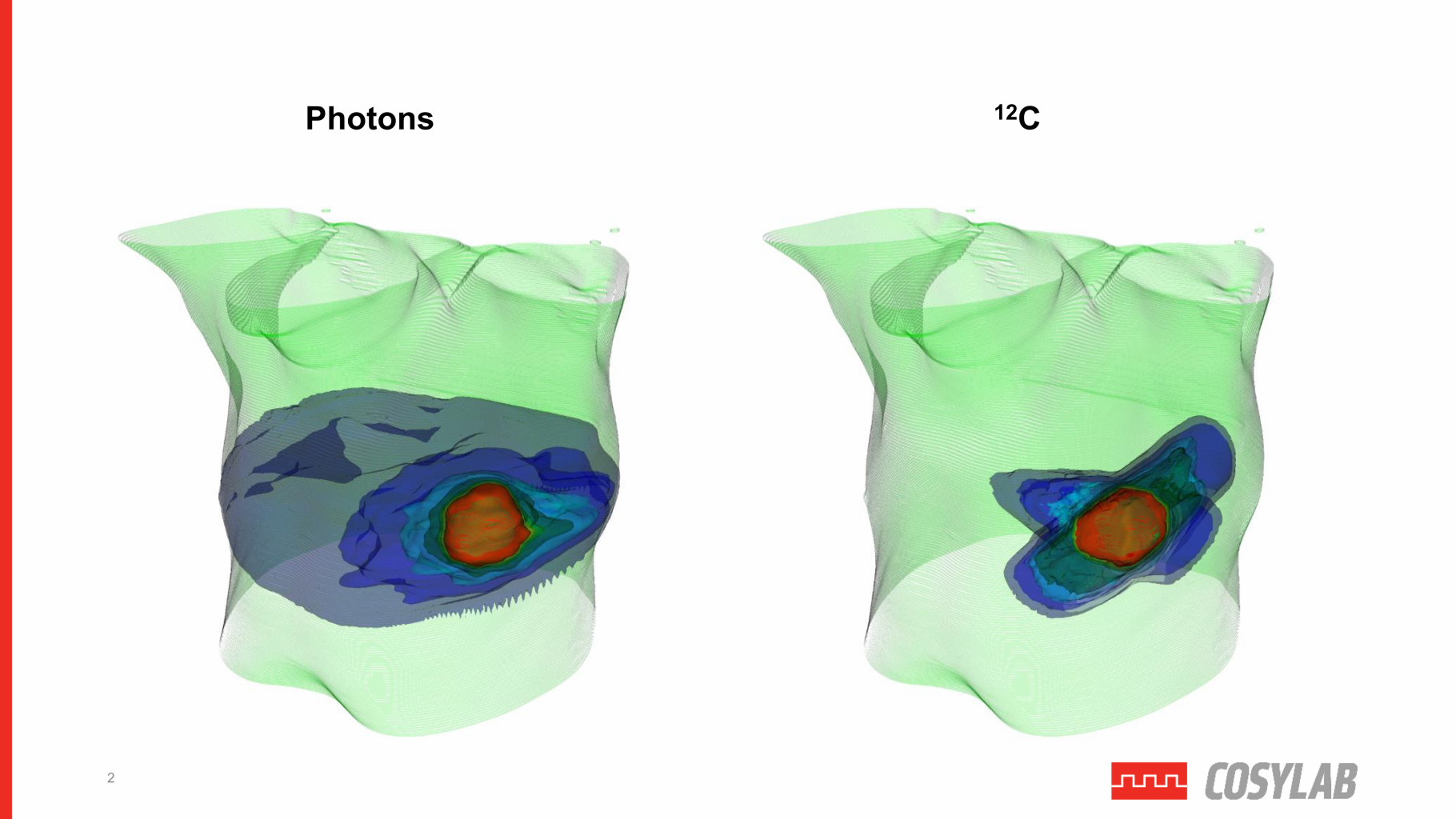
Figure 2 – Represents the difference in irradiated healthy tissue surrounding a tumour between photon therapy and C12 therapy (PT).
The foremost objective of RAPTOR is to reduce the positional uncertainties of the Bragg Peak and generate the PT treatment plan instantaneously, while the patient is on the couch in the treatment room. The plan preparation would be fast, on the fly, so that the patient could even sit or stand during the short process! No additional patient positioning would be required, which now takes about eighty per cent of the whole treatment time.
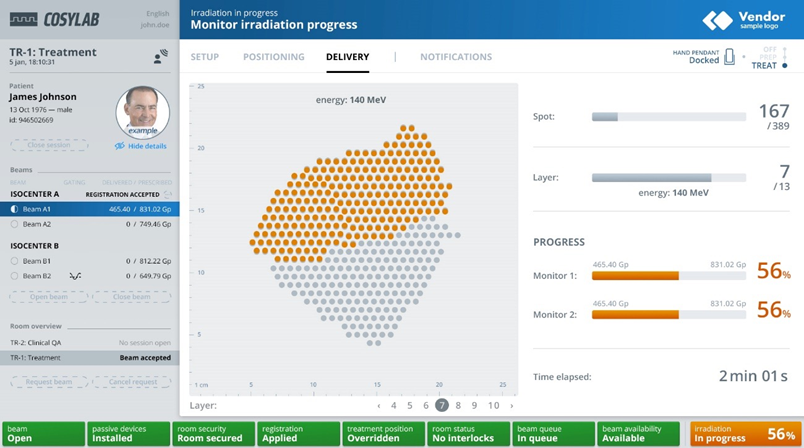
Figure 3 – TreatmentOne is Cosylab’s central workflow manager coordinating all parts of an EBRT system and is future-proofed for RAPTOR-like clinical adaptive PT with dynamic treatment plans.
We can readily imagine the benefits: not only would the patients be more comfortable, but the PT facility would be able to increase patient throughput and lower the cost per session significantly.
SUMMARY
We at Cosylab are proud to be a co-founder and one of the three industrial partners in the RAPTOR EU research project that aims to bring adaptive particle therapy to the clinic. The latter would operate as a practical, real-time system for the rapid preparation of dynamic treatment plans that consider individualistic patient-anatomy changes. The final result will be integrating all the partners’ findings into an innovative technical solution that is both clinic-ready and marketable. I believe proton therapy can become a part of modern value-based healthcare which promises even better cancer-patient outcomes at a cost that society can afford.
The future of particle therapy is not only adaptive – it is also real-time!
This project has received funding from the European Union’s Horizon 2020 research and innovation programme under the Marie Skłodowska-Curie grant agreement No 955956.
This article reflects only the author’s view. The Research Executive Agency and the European Commission are not responsible for any use that may be made of the information it contains.